Technology transfer is a critical process for moving innovative laboratory research to real-world industrial applications. This bridge between discovery and practical use involves numerous challenges, but with the right strategies, these barriers can be overcome to bring breakthrough innovations to market.Â
In this blog, we explore the process of scaling up nanoparticle synthesis for industrial application. Despite the enormous potential of nanoparticles, translating lab-scale successes to commercially viable products is a challenging process that necessitates navigating a variety of technical obstacles, regulatory frameworks, and market dynamics. Let's explore the challenges that researchers and industries must overcome and the strategic solutions that pave the way for a future where nanoparticles make a macroscopic difference.
Challenges in technology transfer

1. Scaling up: One of the primary challenges in technology transfer is scaling up a lab-scale process to industrial production. Processes that work well in a small, controlled environment may not directly translate to larger scales due to issues like changes in reaction kinetics, material properties, or heat and mass transfer.
2. Intellectual Property (IP) management: Protecting the intellectual property generated from research is crucial. Navigating patent law, managing IP rights, and ensuring proprietary technology is not violated during the transfer process can be complex and require extensive legal and technical expertise.
3. Regulatory compliance:Â Meeting industry-specific regulatory requirements is often a significant barrier. Compliance with safety, environmental, and quality standards requires thorough testing and validation, which can be time-consuming and costly.
4. Cultural differences:Â Bridging the gap between the research environment, which often prioritizes exploration and discovery, and the industrial sector, which focuses on efficiency, profitability, and production, can be challenging. These cultural differences can impact communication and project management.
Strategies for effective technology transfer

1. Collaborative partnerships:Â Establishing strong partnerships between research institutions and industry stakeholders can facilitate smoother technology transfer. These partnerships can provide the necessary resources, expertise, and financial support to scale and comply with regulations.
2. Early and continuous engagement:Â Involving potential industrial partners early in the research process helps align the technology development with market needs and industry standards reducing the risk of significant redesigns later.
3. Technology Readiness Levels (TRL):Â Using the TRL framework can help in systematically assessing the maturity of a technology before moving forward with transfer efforts. This assessment helps in identifying risks early and planning the development process to meet industrial needs.
4. Dedicated transfer teams:Â Forming teams focused specifically on technology transfer can enhance the process. These teams, ideally comprising members with expertise in R&D, regulatory affairs, business development, and project management, can address the multifaceted challenges of transferring technology.
5. Incremental scaling and piloting:Â Developing pilot programs or production processes on an incremental scale can identify potential problems early without significant investment. This step-by-step approach helps in mitigating risks associated with direct large-scale implementation.
Practical example - Nanoparticles technology transfer: Challenges and considerations
Nanoparticles are used across a wide range of applications, including medicine, electronics, and materials science, making their large-scale production critical for commercial and industrial use. In the following, we will address the different aspects of nanoparticle technology transfer from lab scale to industrial scale.
Challenges and barriers:
1. Maintaining particle uniformity and quality:
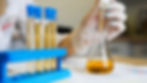
Lab scale: In a laboratory, nanoparticles are typically produced in small batches that allow for precise control over the reaction conditions, leading to high uniformity in particle size and shape.
Industrial scale: Scaling up to larger volumes can disrupt this precision. Issues such as inadequate mixing, temperature gradients, or different rates of reactant addition can lead to variability in particle size and shape, impacting the quality and performance of the nanoparticles.
2. Reproducibility and process control:
Lab scale: Small-scale experiments often benefit from the close monitoring and adjustment of conditions by researchers.
Industrial scale: At an industrial level, the sheer volume of production necessitates automated processes which must be carefully designed to replicate the precise conditions of lab experiments. Ensuring consistent reproducibility in mass production requires sophisticated control systems and sensors to continuously monitor and adjust process parameters.
3. Reaction kinetics and scaling effects:
Lab scale: Reaction kinetics can be directly observed and controlled in a lab setting. Researchers can quickly adjust concentrations, temperatures, and other variables to optimize outcomes.
Industrial scale: When production scales up, the kinetics can change unpredictably. For example, heat transfer becomes more challenging as the scale increases. Exothermic reactions that are easily managed in small reactors may pose significant risks of overheating or even thermal runaway in larger systems.
4. Safety and environmental considerations:
Lab scale: Handling hazardous materials on a small scale involves well-established laboratory safety protocols.
Industrial scale: The same materials at a larger scale can present more risks, including challenges in waste management, emissions control, and worker safety. Industrial setups must incorporate robust safety measures and comply with more stringent environmental regulations.
5. Scalability issues:
Reproducibility at scale: Achieving high yields of nanoparticles consistently is straightforward in the controlled conditions of a lab, where small batches allow for careful monitoring and quick adjustment of synthesis parameters. However, maintaining this yield on an industrial scale can be more challenging due to variations in mixing, heating, and other process conditions that are more difficult to control uniformly across larger volumes.
Process optimization: Techniques optimized for small-scale production may not scale linearly, leading to decreased efficiency or yield when applied to larger batches. For example, issues such as insufficient mixing or heat transfer inefficiencies can lead to incomplete reactions or degradation of the nanoparticles, reducing the overall yield.
6. Economic impact:
Cost-effectiveness: For many applications, the economic viability of nanoparticle-based products depends on achieving high yields. Lower yields at the industrial scale can significantly increase the cost per unit of the nanoparticles, making the technology less competitive in the market.
Material waste: Lower yields also mean higher rates of material waste, which impacts the environmental sustainability of the production process.
7. Quality and performance:
A drop in yield can also reflect variations in the quality and uniformity of the nanoparticles produced. This variability can affect the performance of the final product, particularly in applications where the precise size, shape, and composition of nanoparticles are critical for functionality.
Example: Silver nanoparticles synthesis
Let’s consider the specific example of silver nanoparticles valued for their conductive and antimicrobial properties, making them useful in a variety of applications from electronics to healthcare products.
Lab method:
In the laboratory, silver (Ag) nanoparticles are typically synthesized using a chemical reduction method. Silver nitrate (AgNO₃) acts as the silver precursor, which is reduced in a controlled environment using a reducing agent such as sodium borohydride (NaBH₄). The reaction is usually conducted at room temperature and can be precisely controlled to yield nanoparticles of specific sizes and shapes.
Challenges in scaling up:
Scaling up this process to industrial levels involves several significant challenges:
Maintaining stability and dispersion:Â As the scale of production increases, it becomes more challenging to maintain the stability and dispersion of the nanoparticles. Effective mixing is critical to prevent agglomeration; however, achieving rapid and uniform mixing is more complicated in larger reactors. Poor mixing can lead to the formation of larger or irregular particles, which are less effective and can alter the desired properties of the nanoparticles.
Heat management:Â The reaction between silver nitrate and sodium borohydride is exothermic, releasing a considerable amount of heat. On a laboratory scale, this heat can be easily dissipated. However, in larger volumes, managing this heat becomes more complex. Insufficient heat removal can lead to localized overheating, which can prematurely terminate the reaction or degrade the nanoparticles, affecting both yield and quality.
Yield considerations:
Process efficiency: On a larger scale, the efficiency of the nanoparticle synthesis process can significantly impact the overall yield. Any inefficiencies in the reaction process, such as incomplete reduction or loss of nanoparticles through aggregation, directly reduce the yield.
Economic impact: Lower yields at the industrial scale increase the cost per unit of production. This raises material costs due to more input required for the same output, and increases waste management costs.
Reproducibility: Ensuring that each batch of nanoparticles matches the quality and characteristics of previous batches is crucial. Variability in yield can lead to inconsistent product quality, which is particularly problematic for applications requiring high precision.
Strategic approaches for effective scale-up:
Pilot scale trials: Before full industrial scale-up, conducting multiple pilot scale trials can help in understanding the transition points and critical control parameters necessary for successful large-scale production.
Advanced mixing technology: Employing advanced mixing techniques and equipment that can handle larger volumes while ensuring consistent shear and heat distribution. Techniques like microfluidic mixing or continuous stirred-tank reactors (CSTR) could be explored to enhance the uniformity of the reaction mixture.
Optimized reaction conditions: Fine-tuning the reaction parameters such as the concentration of reactants, temperature, and pH can help maximize the reaction's efficiency and yield.
Real-time monitoring: Integrating sensors and control systems that provide real-time data on critical parameters like temperature, pH, and reactant concentrations to adjust the process instantly.
Applicability of REACH to nanoparticles
1. Registration:Â
Under REACH, manufacturers and importers must register substances, including nanoparticles, if they produce or import them in quantities over one tonne per year. This registration process requires providing detailed information on the substance's properties, uses, and potential health and environmental risks.
2. Specific considerations for nanoparticles:
In 2018, the European Chemicals Agency (ECHA) updated the REACH annexes to include specific provisions for nanomaterials. These changes clarified that nanomaterials need to be treated as separate substances from their non-nanoforms if they exhibit different properties.
Manufacturers and importers are required to provide additional information on particle size distribution, surface treatment, and other physicochemical characteristics unique to nanoparticles.
3. Safety and risk assessments:Â
The safety data sheets must include specific information about the safe handling, transport, and disposal of nanoparticles, reflecting their unique behaviors and potential risks compared to larger particles.
Applicability to nanoparticles-based inks
Nanoparticle-based inks, used in applications like printed electronics and advanced coatings, are also subject to REACH regulation.Â
1. Composition: Nanoparticle-based inks often consist of a carrier medium (like a solvent or polymer) and nanoparticles that provide specific properties (such as conductivity or color). Each component, including the nanoparticles, must be assessed under REACH.
2. Evaluation and authorization: If the nanoparticles or other substances within the inks are identified as substances of very high concern (SVHC), they might be subject to further evaluation and possibly authorization. This is particularly relevant if they pose significant health or environmental risks.
3. Communication in the supply chain: Manufacturers and distributors of nanoparticle-based inks must communicate hazard and safety information to their customers through safety data sheets, ensuring that all parties are aware of any risks associated with the substances and how to handle them safely.
Conclusion
Effective technology transfer requires a strategic approach that addresses the technical, legal, and cultural challenges of moving from the lab to fab. Through collaborative partnerships, early engagement of stakeholders, and methodically managing the scaling up of technology, research institutions and industries can successfully bridge the gap between innovation and commercialization. The challenges in scaling up nanoparticle synthesis are significant—ranging from maintaining strict quality controls to achieving economic viability. However, with the right strategies, such as collaborative partnerships, rigorous pilot testing, and advanced process controls, these obstacles can be overcome and can lead to substantial advancements in a multitude of sectors.
At NETO Innovation, our team is at the heart of navigating these complex transitions. Our expertise in nanomaterials, innovative materials, and technology transfer, makes us experts in the field. We invite you to subscribe to our webpage for more insightful posts like this one. Stay informed about the latest developments in nanotechnology and innovative materials, and learn how strategic technology transfer can enhance both business value and technological impact. Follow us on our social media channels for more informative content like this.  Â

LinkedIn  Â
References:
Robert W. Ciborowski & Iwona Skrodzka. International technology transfer and innovative changes adjustment in EU. Empirical Economics. 59, 1351-1371, 2020. https://doi.org/10.1007/s00181-019-01683-8
Park, W. G. and D. C. Lippoldt (2008), "Technology Transfer and the Economic Implications of the Strengthening of Intellectual Property Rights in Developing Countries", OECD Trade Policy Working Papers, No. 62, OECD Publishing. doi:10.1787/244764462745
Ensuring technology transfer agreements respect competition rules. EUR-Lex, Access to European Union Law. https://eur-lex.europa.eu/EN/legal-content/summary/ensuring-technology-transfer-agreements-respect-competition-rules.html
Nanomaterials 2021, 11(8), 2059; https://doi.org/10.3390/nano11082059
Synthetic Biology, Volume 8, Issue 1, 2023, ysad014, https://doi.org/10.1093/synbio/ysad014Â
Andresen, E., Islam, F., Prinz, C. et al. Assessing the reproducibility and up-scaling of the synthesis of Er,Yb-doped NaYF4-based upconverting nanoparticles and control of size, morphology, and optical properties. Sci Rep 13, 2288 (2023). https://doi.org/10.1038/s41598-023-28875-8
Open Science report "Approaches to scaling up reproducibility in research organisations". SURF - Driving innovation together. https://www.surf.nl/en/news/open-science-report-approaches-to-scaling-up-reproducibility-in-research-organisations
Nanomaterials (Basel). 2021 Sep; 11(9): 2318. Published online 2021 Sep 6. doi: 10.3390/nano11092318
S. Iravani, H. Korbekandi, S.V. Mirmohammadi, and B. Zolfaghari. Synthesis of silver nanoparticles: chemical, physical and biological methods. Res Pharm Sci. 2014 Nov-Dec; 9(6): 385–406.
Konstantia Nathanael, Sibo Cheng, Nina M. Kovalchuk, Rossella Arcucci, Mark J.H. Simmons. Optimization of microfluidic synthesis of silver nanoparticles: A generic approach using machine learning. Chemical Engineering Research and Design. Volume 193, 2023, Pages 65-74, ISSN 0263-8762, https://doi.org/10.1016/j.cherd.2023.03.007.
Zengmin Tang, Woo-Sik Kim, Taekyung Yu, Continuous synthesis of silver plates in a continuous stirring tank reactor (CSTR), Journal of Industrial and Engineering Chemistry, Volume 66, 2018, Pages 411-418, ISSN 1226-086X, https://doi.org/10.1016/j.jiec.2018.06.008.